Introduction
Prenitrogenation is frequently used in out-of-hospital care, hospital setting emergencies, and elective procedures to prepare for the establishment of a secure airway prior to the induction of general anesthesia.1, 2, 3, 4, 5 Prenitrogenation techniques increase oxygen storage, giving vital time to handle an unexpectedly difficult airway—a situation that is not always anticipated. Researchers and medical professionals committed to enhancing patient safety have been debating the most effective ways to achieve prenitrogenation since the mid-1900s.Research comparing prenitrogenation procedures concentrated on several endpoints to assess the effectiveness and efficiency of those approaches. 2, 6 Prenitrogenation aims to reduce any oxygen desaturation that may happen during anesthesia induction and maintain ventilation in a secured airway until ventilation is restored. In the event of severe hypo ventilation or apnea, effective prenitrogenation should lengthen the time until desaturation develops.
Starting from the moment the patient experiences apnea, the Duration of Apnea without Desaturation (DAWD) measure continues until the patient's oxygen saturation falls to less than 90%. This is the point at which oxygen abruptly de-saturates from the hemoglobin molecule, resulting in the onset of hypoxemia. While DAWD can be a highly helpful indicator of a prenitrogenation technique's efficacy in some situations, measuring desaturation time—if purposefully induced—raises ethical questions due to its potential for hypoxemia. Other metrics, such as maximum deep breath volumes that approach vital capacity, end-tidal oxygen concentration, end-tidal nitrogen concentration, and arterial oxygen tension, have been employed as effective surrogates in place of DAWD. 2, 6, 7 In patients breathing 100% oxygen utilizing a minimally leaky breathing apparatus, deep breath volumes approaching a vital capacity breath result in a physiologic enhanced wash-in of oxygen and washout of nitrogen. An elevated oxygen reserve in the lungs was thought to be crucial for the safety of four- and eight-breath prenitrogenation procedures, since it prolongs the time until desaturation in the event of prolonged apnea or hypo ventilation. The efficacy of deep breathing treatments is contingent upon patients attaining deep breathing volumes that roughly correspond to their vital ability to maximally substitute oxygen for nitrogen. Patients' compliance with routine deep breath coaching may be jeopardized if they are under the influence of preoperative sedatives or are otherwise distracted. The purpose of this study is to compare the efficacy of a standard technique with a real-time biofeedback motivating strategy in achieving deep breaths that more closely resemble expected vital capacity in the clinical operating scenario.
Materials and Methods
Prior to entering the operating room on the morning of surgery, patients provided written informed consent to participate in this study, which was authorized by the Institutional Review Board of Manipal University, Bangalore. Patients undergoing elective surgery with an ASA physical status of 1 through 3 were eligible to take part in the trial. A Medline Adult Regular face mask (North-field, Illinois, USA) that had been inflated to improve the interface between the mask and the patient's face, a Medline Anesthesia Circuit (Medline Industries Inc., North-field, Illinois, USA), and a Dräger Apollo® anesthesia machine (Lübeck, Germany) were used for all studies.
For all measurements, the oxygen flow was adjusted at 8 liters per minute and the adjustable pressure-limiting (APL) valve was in the completely open position. Prior to the commencement of the study, patients were randomized into two groups using block randomization: the Investigational group and the Control group. In order to be eligible for participation, patients had to meet certain requirements, which included having a normal capnograph, a Ramsay Sedation Score of less than three during the passive prenitrogenation phase, and a good mask fit as assessed by the investigators. The anesthetic care staff was informed of the patients' randomization status—Control or Investigational—after three minutes of passive prenitrogenation if they satisfied the mask fit and sedation criteria. The investigation was conducted in two stages. One of the investigators gave all of the patients scripted coaching during the first phase, instructing them to "take 4 deep breaths in as deep as you can."
The patient was then made aware of the count by having each breath counted aloud. If the initial deep inhale was less than the anticipated tidal volume, the investigator was allowed to make the request again. In the second phase, the identical initial instruction to take four more deep breaths—as deep as possible—was delivered to the control patients after they had completed the first four. The count was then repeated. On the other hand, real measured volumes (without units) were given to the experimental patients, who were instructed to raise the volume for four more breaths to a goal number of 200 mL over the previous breath. Once the eighth breath was taken, anesthesia was induced. For every breath, the volume and end tidal oxygen were manually recorded.
The pulse oximeter measurements and end tidal carbon dioxide were also recorded. The utilization of video recordings containing capnographic data aided in the confirmation of consistent measuring circumstances for every subject. Patients were placed in the supine position with the OR bed at an angle of 0 degrees for all studies. Under the heading Goal Directed Numeric as a Means of prenitrogenation, this clinical study was registered with clinicaltrials.gov (code CMD24831387). Predicted vital capacity for every patient was determined using the following formulas: the height (cm) for females = (21.78 - 0.101 x (age (yrs)) x height (cm), and the age (yrs) for males = (27.63 - 0.112 x) x (5 cm). The student t-test, Chi square, and Fisher's exact tests were used to evaluate demographic and clinical data between groups. ANOVA with repeated measures was used to evaluate the respiratory and gas exchange parameters. Bonferroni correction was used for multiple pairwise comparisons during post hoc analysis. Software from GraphPad Prism version 9 (San Diego, California, USA) and IBM SPSS version 28 (Chicago, Illinois, USA) were used for statistical analyses. A statistically significant result was defined as a p < 0.05.
Results
To take part in the study, twenty-five patients provided written, informed consent. Three patients were not randomized or otherwise included in the trial because they did not meet the criteria for over-sedation (N = 1) or poor mask fit, which was confirmed by aberrant capnographs suggesting a leak (N = 2). Thus, data analysis was done for two sets of eleven patients (N = 22). Age, gender, BMI, and ASA status of baseline patients were matched equally between the Control and Investigational groups (Table 1).
When it came to intravenous fentanyl premedication, one patient in the Control group received 50 mcg, and two patients in the Investigational group received 50 mcg and 100 mcg of fentanyl before prenitrogenation. Furthermore, 2 mg of midazolam was given to 2 additional Investigational patients, and 7 Control and 8 Investigational patients received 2 mg of the drug. Comparison revealed that there was no difference in these premedication dosages between the groups, and the Ramsey score was 2 for all study participants (N = 22) (Table 1).
Table 1
Demographic information. Based on birth-assigned sex, age and BMI mean and standard deviation, median ASA status, midazolam dose, fentanyl dose, and Ramsay Sedation Scores with representative p-values, the number of patients was determined.
The first four deep breaths (DB) were evoked in both the Control and Investigational groups using the same conventional coaching technique, as described in the Methods section. There was no statistically significant difference between the corresponding DBs in the Control or Investigational subjects when the tidal volumes measured during this prenitrogenation phase were compared (p=0.831, Figure 1). For instance, according to Table 2, the mean volume for DB 4 was roughly 1399 ± 943 mL for the Investigational group and 1286 ± 477 mL for the Control group.
Only the Investigational group used biofeedback motivated approaches for the second four deep breaths.Only the Investigational group used biofeedback motivated approaches for the second four deep breaths. The findings indicate that the Investigational group significantly outperformed the Control group, which received typical coaching approaches, in terms of observed volumes for DB 5 to 8. (p=0.005, Figure 1). Additionally, the investigational DB 8 (mean volume of 2410 ± 859 mL) exceeded the tidal volumes attained by control patients utilizing traditional instructional technique (mean volume of 1267 ± 553 mL) to a p=0.001 (Figure 1, Table 2).
Table 2
Shows the predicted vital capacity (mL) and the analysis of the tidal volumes (mL) for the Control and Investigational groups during deep breaths 1 through 8.
Subsequent calculations concentrated on how closely these DBs increased because the data confirmed that biofeedback-motivated breaths performed during phase two increase mean DB volumes when compared to traditional approach. It calculated the estimated vital capacity (VC) for each patient. A predicted VC (pVC) was determined for every patient by taking into account their gender, height, and age. The two groups' mean pVC volumes did not differ substantially (p=0.393, Table 2). For each patient, the percentage of pVC was determined by dividing the volume attained during each deep breath by the patient-specific projected VC.
Figure 2 displayed the means and standard deviations of these findings. . The % change from pVC for standard DB 1 through 4 did not significantly differ between the Control and Investigational participants, according to the results (p=0.477). Furthermore, there was no variation in the percentage of pVC volumes for every database run by the Control group. However, when compared to ordinary DBs, biofeedback-coached DBs 5 to 8 approached pVC more closely (p=0.002). For instance, DB 8 was just 39.37 ± 15.54% of pVC for the Control group (p<0.001), whereas it was almost 70.45 ± 16.48% of pVC on average for the Investigational group.
Figure 1
For the Control and Investigational groups, the deep breath volumes (mL) mean and standard deviation are provided. For both groups, the two-phase technique is demonstrated. The left panel displays the first four deep breaths that were evoked using the conventional method, while the right panel displays the next four deep breaths that were observed using the experimental biofeedback methodology. In comparison to the Control subjects (blue circle), the Investigational subjects (red square) who received coaching showed a statistically significant increase in deep breath volumes throughout phase two. P-values show a substantial difference between the four conventional DBs and the coached DBs 6–8.
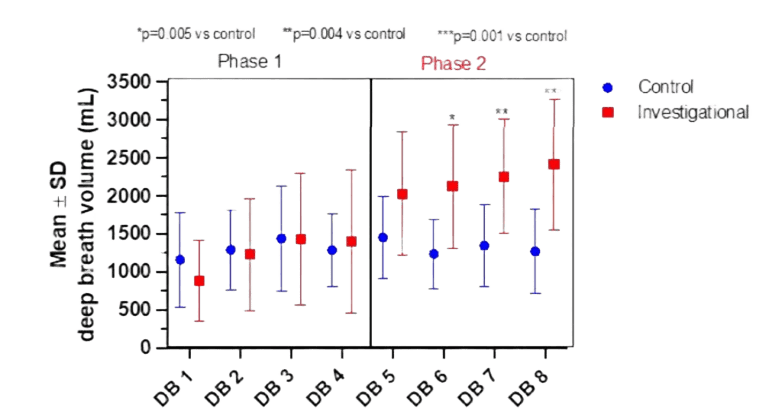
Figure 2
The % difference from the expected vital capacity for the Control and Investigational groups, together with the mean and standard deviation. According to the Methods section, the volume at each DB and each patient's computed VC are represented by the percent change from pVC. The results from phase 2, when the biofeedback motivated technique was utilized only for the Investigational group, was displayed in the right panel, and percentages obtained from volumes measured from phase 1 of the protocol, where traditional techniques were employed for both groups, were presented in the left panel. In comparison to the Control individuals (blue circle), the p values show a statistically significant increase in the percent shift from expected vital capacity in the Investigational subjects who were taught to conduct deep breaths (DB 5 to 8) utilizing biofeedback (red square).
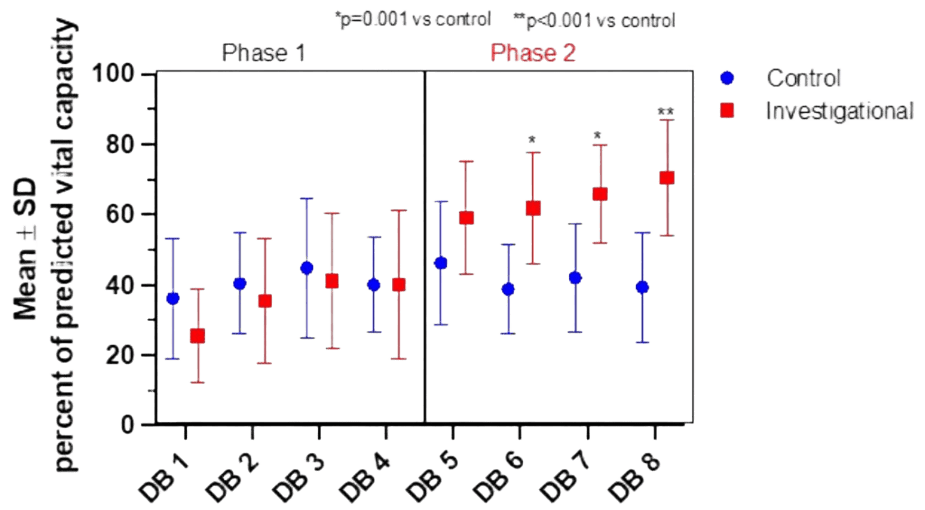
Figure 3
It shows the mean and standard deviation of the EtO2 levels for the Control and Investigative individuals during the two-phase experiment. The mean EtO2 is displayed using bar graphs in mmHg for the experimental subjects (in red) and controls (in blue). The significance between DB 5 and 8 for the Controls, DB 5 and 8 for the Investigational group, and DB 6 and 8 for the Investigational group was indicated by the p-values that were reported.
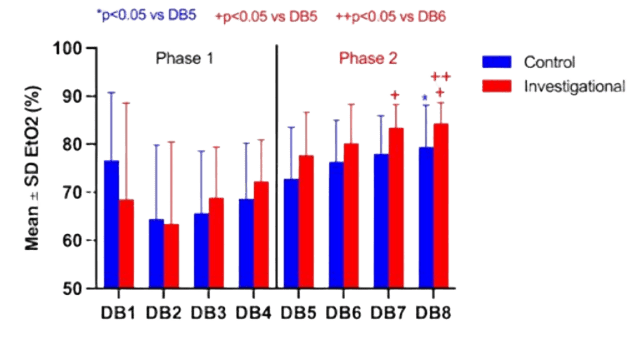
Table 3
It shows the mean and standard deviation of the end-tidal oxygen levels for the Phase 2 Control and Investigative groups. EtO2 was measured and reported in mmHg. After taking a deep breath. The one-sided student paired t-test was used to compare means.
|
Control N=1 |
Investigational N=11 |
p-value |
|
|
End tidal oxygen (mm Hg, Mean ± SD ) |
|
|
|
48.7 ± 12.6, 44.4 ± 11.7 |
0.523 |
DB5 |
73 ± 11 |
78 ±9 |
0.13 |
|
|
DB6 |
76± 9 |
80± 8 |
0.15 |
|
|
DB7 |
78± 8 |
83 ±5 |
0.03 |
|
|
DB8 |
79 ±9 |
84 ±4 |
0,05 |
|
|
The end-tidal carbon dioxide (ETCO2), end-tidal oxygen (EtO2), and average oxygen saturation that were measured at the conclusion of each database were then examined. Between the Control and Investigational groups, there was no difference in oxygen saturation or ETCO2 as determined by a pulse oximeter. Furthermore, during prenitrogenation, induction, or intubation, no desaturations—defined as an oxygen saturation below 95%—were noticed. No statistical The end-tidal carbon dioxide (ETCO2), end-tidal oxygen (EtO2), and average oxygen saturation that were measured at the conclusion of each DB were then examined. Between the Control and Investigational groups, there was no difference in oxygen saturation or ETCO2 as determined by a pulse oximeter. Furthermore, during prenitrogenation, induction, or intubation, no desaturations—defined as an oxygen saturation below 95%—were noticed. For DB 1 through 4, there was no statistically significant difference in recorded EtO2 between Control and Investigational participants (p=0.905); however, this is not surprising because the oxygen saturation was still greater than or equal to 96% before mask ventilation. Nevertheless, mean EtO2 did considerably rise for DB 7 and 8 in the later portion of phase 2 (Table 3). In both groups, post hoc analysis revealed a significant within-group rise in EtO2 from DB 5 to 8 (Figure 3). Regarding the Research group, EtO2 levels at DBs 7 and 8 were considerably higher than those at DB 5 (p=0.029, p=0.014). A significant difference was also seen between the mean at EtO2 DB 8 (84.4 ± 3.6 mmHg) and DB 6 (80.2 ± 6.5 mmHg, p=0.027).
Discussion
The authors of this study postulated that using real-time, goal-directed coaching to encourage greater volume deep breaths than typical coaching strategies could enhance maximal deep breaths during prenitrogenation. When compared to the Control individuals, this study clearly showed that the Investigational subjects who were taught to do four biofeedback deep breaths had a statistically significant increase in deep breath volume that more closely matched projected vital capacity.Three elements make up Dr. McClelland's motivational theory: (1) a clear, attainable goal; (2) prompt feedback; and (3) an appropriate reward. The objective of this study is for the patient to inhale more deeply than before, and the volume of each breath given at the conclusion provides instant feedback. The patient's efforts will pay off in the form of improved overall care. The application of these methods takes weeks or years in conventional research, however in this study, biofeedback deep breath coaching was effectively used for minutes before induction. Each subject received a uniform script to enable data comparison.
All research participants were advised that prenitrogenation with a face mask enhances anesthetic safety prior to induction. It's interesting to note that this "reward" was insufficient on its own to encourage patients to take their deepest breath possible in either the first or second phase for control patients. Every practitioner told the patient to "take 4 deep breaths in as deep as you can" during the first mask ventilation phase. The patient was given instructions to raise each breath volume by 200 and each breath volume was recited to them during the second phase, or (1) the previous instructions were reiterated.
This particular wording was chosen by the researchers because it places greater emphasis on maximal inspiratory effort than expiratory effort. A large breath volume attained through expansion is more likely to maintain the integrity of the narrow airway than a deep breath that is accomplished by raising the expiratory reserve volume (ERV) and possibly surpassing closure capacity (CC) in patients at higher risk. Regretfully, it was impossible to ascertain if patients were more driven to raise IRV than ERV in an attempt to get a higher figure. Patients in the experimental group were given a new goal volume that was marginally larger than the baseline and were orally given a straightforward numerical value that was exactly as it appeared on the anesthetic machine monitor. The size of their previous breath to give them real-time information about their health in real time. The prenitrogenation sequence's short duration and the requirement for breath-by-breath data made it impossible to provide anything more than a straightforward number. The straightforward numerical data was adequate to independently promote a rise in deep breath volume in the patients in the investigational group, despite its lack of clarity due to the absence of units and other context, such as the optimal value they could achieve. End-tidal oxygen acted as a proxy, as evidenced by multiple studies that found that end-tidal oxygen levels above 85% had less desaturation occurrences in a range of clinical contexts. The indicator of sufficient arterial prenitrogenation. Since the Investigational group began with four deep breaths using traditional coaching in the same way as the Control group, the study was not intended to identify changes in end tidal oxygen concentration between groups. However, there was evidence of a statistically significant difference between the Control and Investigational groups' expired oxygen concentrations for deep breaths 7 and 8. It could be interpreted as a therapeutic advantage. A bigger group of patients receiving only biofeedback coaching as opposed to only standardized coaching might have had higher clinical and statistical significance. Using the proven biofeedback-driven approach to effectively increase the functional residual capacity (FRC) oxygen stores could improve patient care, particularly for critically ill patients or in emergency situations.
It is well known that minute ventilation rises with increasing lung capacities. Increased ventilation causes the blood to become more alkaline and binds oxygen to hemoglobin more firmly, which causes the hemoglobin saturation to shift to the left and cause a slower hemoglobin
desaturation. 1, 3, 4 Although the study groups' arterial partial pressure of oxygen (PaO2) was determined to be identical, Baraka et al. concluded that eight deep breaths were a better approach than the three-minute TV technique. This protective mechanism was suggested as an explanation for their findings. 3, 4, 8 The amount of oxygen stored in the FRC is the main factor influencing total body oxygen intake, which rises exponentially and reaches a plateau after one minute.3, 4, 9, 10, 11 Blood oxygen, on the other hand, contributes significantly less to the body's overall intake and plateaus virtually instantly. Finally, tissue oxygen stores continue to rise gradually in a linear pattern. to rise over a number of minutes, which is why three to five minutes of prenitrogenation are beneficial.5, 9 As anticipated, there were no discernible variations in ETCO2 between the Investigational and Control groups. This is probably because the Investigational group experienced enhanced alveolar sampling of ETCO2 (increased ETCO2) and higher minute breathing (decreased ETCO2).
The short lag time between the patient's actual deep breath and the volume shown on the anesthetic machine is another drawback of the study approach, making it more challenging to offer prompt information about a patient's fast breathing. Although this was not a problem in our study, it is likely to come up in clinical practice at some point, thus the method employed here might not work for such patients. The simplest way to illustrate the variations in prenitrogenation methods would be to measure DAWD or another oxygen tension measurement with a predetermined apneic period, as observed in observational studies conducted in emergency situations.12 Measuring the time it took for patients in our elective patient research to reach hypoxemic states may have put study participants at needless risk, which could present ethical issues. Last but not least, coaching may expose unblinded researchers to bias in volume evaluations; however, this worry was initially allayed by treating the Control and Investigational groups similarly for the first four breaths of the study. Without any proof in phase 1, it would be more challenging to induce investigator bias in phase 2.
Conclusion
In conclusion, this study showed that a biofeedback-driven prenitrogenation regimen is more effective than a conventional coaching method at boosting the volume of deep breaths. Future studies employing this motivational tool may aim to show increased prenitrogenation efficacy, but the degree to which it increased the patient's deep breath volume points to a different line of inquiry. In order to identify patients who are more likely to experience perioperative pulmonary issues that were not detected before to surgery and who would benefit from a different approach like non-invasive ventilation, it might be more beneficial to concentrate on those who do not respond to this motivational tool. 13 It may be possible to develop artificial intelligence-driven processing software that can quickly process respiratory performance data from patients and provide more timely feedback for attainable objectives. 14, 15